Online Tool for 3D Visualization of Gene Mutations
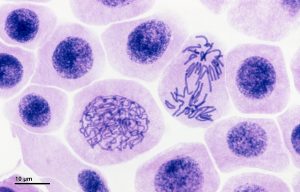
Fifteen years ago marked a major milestone in the Human Genome Project: scientists successfully sequenced all of the base pairs in our 23 sets of chromosomes. Following this accomplishment, researchers assembled generations of mathematical models to understand how gene mutations result in disease. A key barrier in developing these models is the size of genome itself: a single human genome requires approximately 2 GB of storage, and many studies examine thousands of genomes to detect changes in a small number of patients. Both processing these large datasets and efficiently storing them create challenges. Making these model predictions accurate and complete is another challenge.
Scientists collaborating among several universities on three continents developed an online computational tool to help overcome these barriers. The scientists, who include Bernhard Palsson, Ph.D., Galletti Professor of Bioengineering at University of California, San Diego, as one of the lead authors, report on the resource in a recent issue of Nature Biotechnology.
Called Recon3D, the new resource provides a metabolic network model using approximately 17% of known human genes. The model combines data on the genes, metabolites, proteins, and metabolic reactions for human metabolism. In addition, as the model’s name implies, Recon3D accounts for the physical structure of model components, imporving significantly on past models that relied on linear, two-dimensional models. Although the model still has 83% of genes left to incorporate, it could ultimately unravel some of the mysteries underlying virtually any disease with a genetic cause, from inborn errors of metabolism to cancer.
Bioengineering for Refugees
As the war in Syria enters its seventh year, at least five million refugees have left the country to seek asylum elsewhere. Roughly 20% of the refugees are now in Lebanon, where many reside in refugee camps. Although these refugees are now much safer than before, even in the best of circumstances, the conditions in refugee camps can compromise health and wellness.
Engineering can offer relief for some of these conditions. A three-week course offered in January at the American University of Beirut, co-designed and taught by Muhammad Zaman, Ph.D., Professor of Biomedical Engineering at Boston University, and entitled “Humanitarian Engineering: Designing Solutions for Health Challenges in Crises,” had students devising solutions to the issues facing these refugees.
Among the ideas generated by the students was “3D Safe Water” – a device designed to detect the contamination of water, decontaminate it, and deploy the technology in low resource settings. The device uses sensors to detect contamination and chlorine to decontaminate. With water-borne diseases taking an especially hard toll on camps like these, the device could significantly improve living conditions for refugees.
Placenta on a Chip
Organ-on-chip technologies use microfluidics to model organs or organ systems. So far, engineers have developed chip-based models of the lungs, heart, and kidneys, as well as the circulatory system.
The most recent addition to the organ-on-chip family is the placenta-on-a-chip, developed by Dan Huh, Ph.D., Wilf Family Term Assistant Professor of Bioengineering at the University of Pennsylvania. Modeling the organ that mediates and communicates between a pregnant woman and the fetus, Dr. Huh created a chip to study how drugs move from the bloodstream of the mother to the fetus. With this knowledge, one could determine more safely and more accurately how drugs taken by the mother can affect a pregnancy.
People and Places
Two colleges have announced new biomedical engineering programs. George Fox University, a Christian college in Oregon, will offer a BME concentration for engineering majors starting this fall. On the other side of the country, Springfield Technical Community College in western Massachusetts will offer a two-year associate’s degree in BME technology.
The University of Arizona, in cooperation with the City of Phoenix, will launch a new medical technology accelerator program, to be called InnoVention. It will be located on UofA’s Phoenix Biomedical Campus. Frederic Zenhausern, PhD, MBA, Professor of Basic Medical Sciences and Director of the Center for Applied NanoBioscience and Medicine at Arizona, is among the people leading the effort.
Finally, Distinguished Professor Craig Simmons of the University of Toronto’s Institute of Biomaterials and Biomedical Engineering is among 10 awardees sharing a $3.5 million grant (approximately $2.7 million in U.S. currency) for the development of medical devices and technologies. Dr. Simmons, a former postdoc at Penn, will use his funding to investigate the use of stem cells to repair congenital heart defects in infants.