Live Bone Cells Grown in Lab
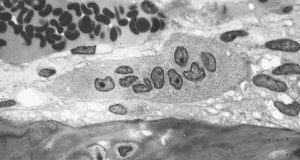
Bone injuries and bone loss can constitute major challenges for patients and the people who treat them. Beyond the need for bone grafts or artificial implants in cases such as severe fractures, cancers metastasizing to the bones can be disabling and disfiguring. Doctors are able to use autologous bone grafts, in which patients are their own bone donors and provides grafts from other bones in their bodies. However, the grafting process compromises the bone from the donor site. In addition, there are specific problems in cases of long bones, such as those in the arms and legs. With these bones, no site of the body can provide sufficient material without becoming severely compromised itself due to bone loss.
Stem cells have been intensively investigated as a source of bone grafts. With their ability to produce a variety of cell lines from the same source, these cells have the potential to be used in a variety of clinical situations. The mechanisms underlying the determination of the type of cell that an individual stem cell will become are known. However, the ability to produce living bone cells in the laboratory had remained elusive – until now. In an article published online last week by Nature Biomedical Engineering, a group of scientists led in part by Professor Matthew Dalby, a cellular engineer with the Institute of Molecular, Cell and Systems Biology at the University of Glasgow, United Kingdom, reported its success.
Professor Dalby’s tissue engineering team used a nanoscale bioreactor to stimulate mesenchymal stem cells into osteogenesis (bone creation). The bioreactor applied vibrations on a microscopic scale of 1,000 hertz with 15 nanometers of vertical displacement. In their previous work, Professor Dalby and his colleagues could generate only one bone cell sample at a time. In the current paper, they showed the ability to generate multiple cells for three-dimensional tissue. In addition, they showed that the cells could be generated in environments with less rigidity than that in which osteogenesis normally occurs. This is an important advance because the body provides optimal conditions of stiffness for this process, but the lab does not. Should the techniques in the paper prove viable on a greater scale, they could revolutionize the field of bone grafting.
Microfluidics in the News
Since their introduction, organs on a chip (OOCs) have proliferated in the field of bioengineering. These chips use microfluidics technology to create a model of an organ system in the body. However, until now, OOCs have not been used to model the human placenta – the tissue that connects the embryonic sac to the uterine wall during pregnancy.
Responding to the lack of a OOC model of the placenta, two professors at Florida International University (FAU) have developed a placenta OOC. Sarah E. Du, Ph.D., assistant professor of ocean and mechanical engineering, and Andrew Oleinkov, Ph.D., associate professor of biomedical science, have collaborated to create this chip, which they to intend to use to determine the effects of malaria on the placental microenvironment. A $400,000 grant from the NIH will certainly help.
With malaria causing more than 200,000 perinatal deaths annually, beyond the burden we cited last week, there is an urgent need to determine the exact effects of this parasitic infection on the placenta. Without this knowledge, the development of technologies to mitigate or even prevent these effects will be much more difficult. In addition, because of the obvious ethical constraints on prospective testing in natural history studies, the placenta OOC offers an ideal model.
Elsewhere in the field of microfluidics, an NIH grant to scientists at the University of Illinois, Urbana-Champaign, has gone toward the development of a new test chip to detect sepsis, a condition in which the body’s reaction to infection results in inflammation of the blood vessels and which can cause lethal shock unless detected and treated promptly. The UIUC team developing this more rapid diagnostic technology is led by Rashid Bashir, Ph.D., professor of bioengineering and associate dean of UIUC’s Carle Illinois College of Medicine. Dr. Bashir was lead author on a paper published over the summer in Nature Communications.
Among the more remarkable aspects of the chip developed by Professor Bashir and his colleagues is that it can diagnose sepsis with a single drop of blood. Therefore, in addition to the device’s portability and size, which allows it to be used at the point of care, it is only necessary to use 10 microliters of blood to complete the test. Other available lab tests for sepsis can require as much as 300 times as much blood. Testing its device against the gold standard of flow cytometry, the UIUC team found that the findings obtained with its biochip were strongly correlated with those from flow cytometry. Unlike the new chip, flow cytometry cannot be performed outside the lab.
Since a large proportion of sepsis patients are treated in intensive care units, the ICU is a likely setting in which the biochip could be used, particularly because some ICUs might be in hospitals where the staff does not have 24-hour lab access. The ability to use this chip at the bedside immediately, rather than waiting until the next morning or longer, could make a key difference in detecting and treating sepsis.
Brains on the Internet
For years, Ray Kurzweil, the computer scientist turned author and inventor, has been discussing a future in which, he claims, the distinction between human and artificial intelligence will disappear. For example, Kurweil imagines brains being uploaded to computers. While what Kurzeil imagines has yet to materialize, scientists in South Africa have created the “Brainternet,” which streams brain waves onto the Internet in real time.
As a student project at the School of Electrical and Information Engineering of the University of Witerstand in Johannesburg led by Adam Pantanowitz, a lecturer in the school, the Brainternet was developed from pre-existing technology. The project starts with portable electroencephalography (EEG), which is worn by the subject and which transmits its signal by telemetry to a Raspberry Pi computer. Then, using open source software, the computer live streams the data to an application programming interface, which in turn allows the data to be published at a website accessible to others.
Beyond being an innovative use of these technologies, the Brainternet could be used in telemedicine applications. For instance, it could be helpful in situations where a specialist neurologist is not in the immediate geographic vicinity. Moreover, for research projects involving EEG measurement during tasks or under certain types of external stimulation, the Brainternet could allow for a much larger sample size to be enrolled, owing to its portability and use of the Internet.
People and Places
Dawn Elliott, Ph.D., chair of the Department of Biomedical Engineering at the University of Delaware, has been elected president of the Biomedical Engineering Society (BMES), for which she had served as treasurer. Dr. Elliott’s term as president will begin in October 2018 and last for two years. As president, she plans to take a closer look at education in the field to determine how bioengineering and biomedical engineering departments can graduate the most successful students. We wish her the best of luck and hearty congratulations.