The Solomon R. Pollack Award for Excellence in Graduate Bioengineering Research is given annually to the most deserving Bioengineering graduate students who have successfully completed research that is original and recognized as being at the forefront of their field. This year, the Department of Bioengineering at the University of Pennsylvania is proud to recognize the work of four outstanding graduates in Bioengineering: William Benman, Alex Chan, Rohan Palanki and Sunghee Estelle Park.
Read more about the 2024 Solomon R. Pollack awardees and their doctoral research below.
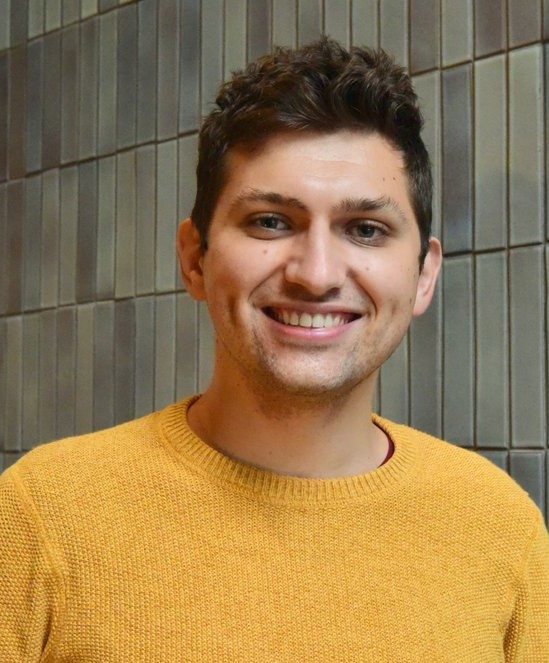
William Benman
Dissertation: “Remote control of cell function using heat and light as inputs”
Will conducts research in the lab of Lukasz Bugaj, Assistant Professor in Bioengineering, focusing on reprogramming cells so that their basic functions can be regulated artificially using heat and/or light as inputs. The goal of this work ranges from clinical applications, such as localized activation of cell therapies within patients via application of heat, to biological manufacturing, using light to activate production of valuable biologics during key phases of a cell’s life cycle. He earned his undergraduate degree in biomedical engineering from Boston University, where he graduated summa cum laude. At BU, he worked in the lab of Wilson Wong, where he was introduced to synthetic biology. During that time, he worked to develop a genetic logic framework that would allow cells to integrate chemical signals, such that each combination of signals would lead to a different, user-defined combination of genes being expressed. Outside of the lab, Benman enjoys baking and sharing his treats with lab members. He mentored the 2021 Penn iGEM team, which recently published their work in Communications Biology. After graduation, he will start a postdoctoral fellowship in Mikhail Shapiro’s lab at Caltech, where he plans to explore electrogenetics, focusing on how to co-opt electrically active cell types to transmit biochemical information out of the body. He is interested in researching ways to get cells to talk to electronic devices and vice/versa for two way communication, especially in the context of patient monitoring and precision therapies.
“Will’s Ph.D. work broke new ground across several fields, discovering how certain proteins sense temperature, engineering those proteins for on-demand control of human cells, and building devices to allow us to communicate with cells with precision,” says Bugaj. “He has managed these accomplishments while elevating those around him through mentorship, including of graduate students, scores of undergraduates, and even grade-school students in the community. I am immensely proud of Will and what he has accomplished and am gratified by the recognition from the Sol Pollack award.”

Alex Chan
Dissertation: “Engineering small protein based inhibitors and biodegraders for cytosolic delivery and targeting of the undruggable proteome”
Alex conducts research in the lab of Andrew Tsourkas, Professor in Bioengineering and Co-Director, Center for Targeted Therapeutics and Translational Nanomedicine (CT3N). His research focuses on developing novel cancer therapeutics by engineering protein scaffolds so that they can be efficiently delivered into cells using lipid nanocarriers. These proteins can either behave as oncogenic inhibitors or be imbued with E3 domains for targeted protein degradation. He graduated from The Pennsylvania State University in 2018 with a B.S in Biomedical Engineering. There, he conducted undergraduate research on photo-activated silver nanoparticle miRNA delivery systems and wrote his senior honors thesis on this topic. At Penn, Alex served as a wellness co-chair within GABE (the Graduate Association of Bioengineers) and was awarded a graduate research fellowship program award by the National Science Foundation (NSF GRFP). In his spare time, Chan loves to cook and explore the local restaurant scene (and he thinks Philly is one of the most vibrant food meccas in America). Post-graduation, he plans to explore Asia before starting as a Senior Scientist in the biopharma industry. He intends to continue working on novel biologics-based medicines for unmet medical needs.
“I cannot think of anyone more deserving of this award than Alex,” says Tsourkas. “He not only demonstrates all of the traits that we love to see in our most successful Ph.D. students — intelligence, hard work ethic, and perseverance — but Alex has also exhibited a level of scientific independence that is beyond his years. I cannot wait to see what Alex achieves in the future.”

Rohan Palanki
Dissertation: “Ionizable lipid nanoparticles for in utero gene editing of congenital disease”
Rohan completed his B.S. in Bioengineering from Rice University in 2019 and subsequently matriculated into the Medical Scientist Training Program (M.D./Ph.D.) at the University of Pennsylvania. He conducted his doctoral research as an NIH Ruth L. Kirschstein Pre-Doctoral Fellow in the laboratories of Michael J. Mitchell, Associate Professor in Bioengineering, and William H. Peranteau, Associate Professor of Surgery at CHOP. After defending his thesis in 2024, he returned to medical school to complete his clinical training. He plans to pursue a career as a physician-engineer, conducting translational research at the intersection of biomaterials and genomic medicine. Outside of the lab, Palanki enjoys exploring new restaurants in Philadelphia and cheering on Philadelphia sports teams.
“Rohan pioneered new lipid nanoparticle gene editing technology in the lab that can treat deadly childhood diseases before a child is ever born,” says Mitchell. “Rohan is extremely deserving of this award, and I cannot wait to see what he accomplishes as a physician scientist developing new biomaterial and drug delivery technologies for pediatric applications.”
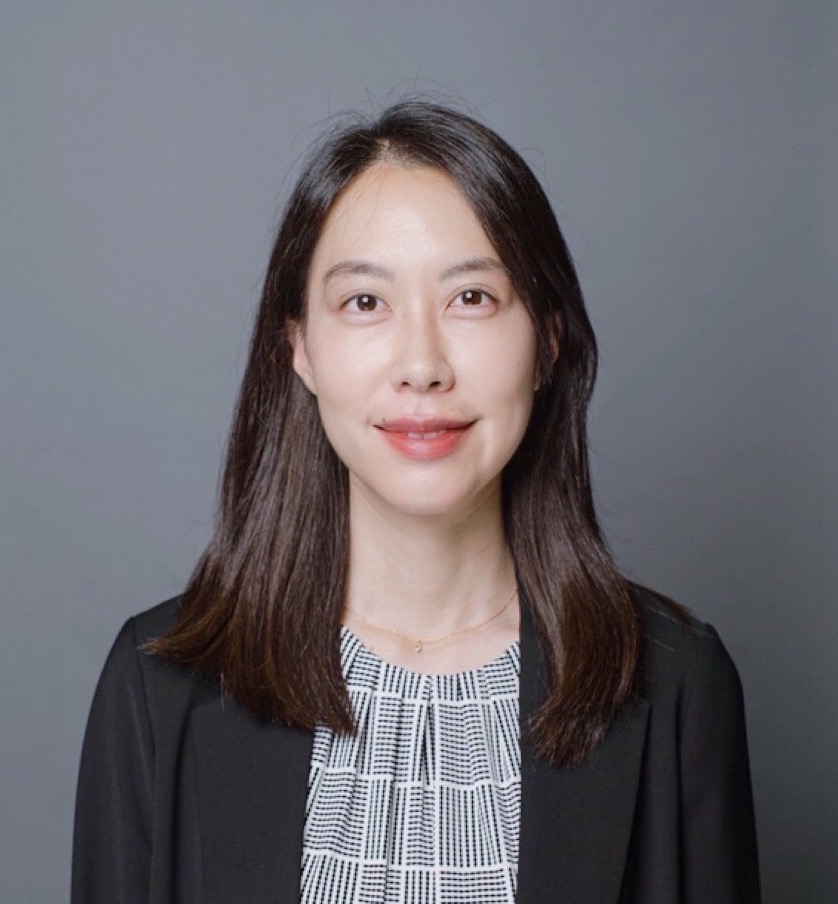
Sunghee Estelle Park
Dissertation: “Engineering stem cells and organoids on a chip for the study of human health and disease”
Sunghee Estelle Park earned her BMSE and MSME from Korea University and her Ph.D. in Bioengineering at the University of Pennsylvania, graduating in July 2023. She conducted doctoral research in the BIOLines Lab of Dan Huh, Associate Professor in Bioengineering. Her Ph.D. research combined principles in developmental biology, stem cell biology, organoids, and organ-on-a-chip technology to develop innovative in vitro models that can faithfully replicate the pathophysiology of various human diseases. Her doctoral dissertation presented engineering approaches to create stem cell derived three-dimensional (3D) miniature models of human organs on a chip that mimic the physiology and function of living human tissues. Park was appointed Assistant Professor of Biomedical Engineering in the Weldon School of Biomedical Engineering at Purdue University beginning January 2024. Her research lab focuses on using engineered tissues and organoid models to understand how biomechanical and biochemical cues direct stem cell differentiation, maturation, and function during development and disease progression, with a particular emphasis on the lung and intestine.
“With her deep knowledge, extensive experience, and leadership, Estelle led the major undertaking of harnessing the power of microengineering technologies to create more in vivo-like culture environments in my group, and she played a central role in demonstrating the proof-of-concept of generating organoid-based in vitro models that enable new capabilities for studying complex human diseases and developing new therapeutics,” says Huh. “I am extremely proud of her tremendous accomplishments as a trailblazer in this emerging area and have every confidence that her work as an independent investigator will continue to make great contributions to advancing the field.”