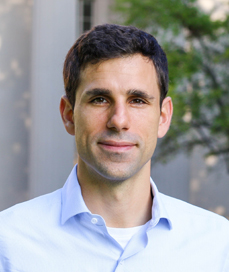
The impending danger of bacterial resistance to antibiotics is well-documented within the scientific community. Bacteria are the most efficient evolvers, and their ability to develop tolerance to drugs, in addition to antibiotic overuse and misuse, means that researchers have had to get particularly resourceful to ensure the future of modern medicine.
Presidential Assistant Professor in Bioengineering, Microbiology, Psychiatry, and Chemical and Biomolecular Engineering César de la Fuente and his team are using an algorithm to search the human genome for microbe-fighting peptides. So far, the team has synthesized roughly 55 peptides that, when tested against popular drug-resistant microbes such as the germ responsible for staph infections, have proven to prevent bacteria from replicating.
WIRED’s Max G. Levy recently spoke with de la Fuente and postdoctoral researcher and study collaborator Marcelo Torres about the urgency of the team’s work, and why developing these solutions is critical to the survival of civilization as we know it. The team’s algorithm, based on pattern recognition software used to analyze images, makes an otherwise insurmountable feat tangible.
De la Fuente’s lab specializes in using AI to discover and design new drugs. Rather than making some all-new peptide molecules that fit the bill, they hypothesized that an algorithm could use machine learning to winnow down the huge repository of natural peptide sequences in the human proteome into a select few candidates.
“We know those patterns—the multiple patterns—that we’re looking for,” says de la Fuente. “So that allows us to use the algorithm as a search function.”
Read Max G. Levy’s “An AI Finds Superbug-Killing Potential in Human Proteins” at WIRED.
This story previously appeared in Penn Engineering Today.