Throughout the Spring 2017 semester, our professor, Dr. David Issadore, taught us (a class of eight undergraduates students and one graduate student) about microfluidics and point-of-care diagnostics. The next phase of the course was to come up with a new diagnostic for pediatric tuberculosis. At the end of the semester, our final assignments included submitting an NIH Research Project Grant (R01) proposal and giving a 20-minute presentation for our devices. These assignments greatly prepared us for our trip to Ghana, as we were able to ask questions and get feedback on our proposed devices by speaking to healthcare professionals at Ghanaian hospitals, clinics, and research facilities. The semester course was mainly focused on the technical design of our devices, which enabled us to hone in on the practical and real-world implementation of the devices while in Ghana. This week, the BE Blog will publish our summaries.
Fecal Diagnostics for Pediatric Tuberculosis
Katharine Cocherl ’20, Kathleen Givan ’20, Kaila Helm ’20, Hope McMahon ’18, David Pontoriero ’18
In order to address the numerous diagnostic problems specific to pediatric tuberculosis in low-resource settings, we have designed a device that uses a fecal sample rather than the current method of sputum samples. Because many children cannot produce sputum samples with the required quality and quantity of sputum, we decided to use stool samples. This noninvasive substitute will ideally allow us to collect all the bacteria swallowed by the patient. The bacterium that causes the disease, Mycobacterium tuberculosis (MTB), is very hardy and has been found to appear in fecal matter. However, this method may be difficult because there are many other substances in fecal matter that need to be removed. By filtering out these impurities, the presence of the bacteria can be detected.
The device we designed is essentially a disposable cartridge that separates virulent TB bacteria from all other fecal material. This collection can be performed with no power and minimal technician input and can be obtained in any desired volume. The total operation time is predicted to be 90 minutes.
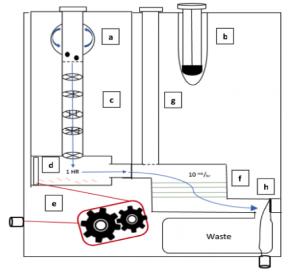
The first aim of our project is to identify a target protein on the surface of the bacteria so that the bacteria can be isolated from the solution. Next, the MTB will be enriched from fecal samples with a single-use filtration device so that a final sample can be provided in a similar form as a sputum sample. This final sample can then be used for smear microscopy, in which technicians look for the presence of the bacteria under a microscope, or for use with the GeneXpert. The GeneXpert is an automated diagnostic test that can identify MTB DNA and resistance to the most potent TB drug, rifampicin. These devices have been distributed to labs and hospitals across Ghana, but they are not yet widely used for general diagnostics.
Because the number of GeneXperts available and the infrastructure supporting them are increasing, we are hopeful that, in the near future, our diagnostic will be able to be used in conjunction with this technology. Upon integration with the GeneXpert system, our device would be able to increase sample specificity for the underserved demographic of pediatric TB patients. In addition, as technology becomes available in smaller, more local clinics, we foresee lower travel burdens for families and lower operational costs for healthcare facilities.
We are beyond grateful for the opportunity to engage with Ghana’s medical system. Before traveling to Ghana, we created a proposal for our fecal diagnostic for pediatric TB. After learning more about the current medical system and infrastructure in place, we were able to revise our ideas in a meaningful way. It is our hope that one day a project of this magnitude can come to fruition.