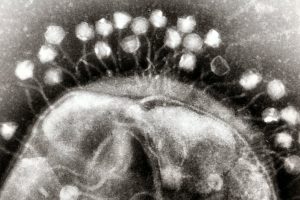
Before CRISPR became a household name as a tool for gene editing, researchers had been studying this unique family of DNA sequences and its role in the bacterial immune response to viruses. The region of the bacterial genome known as the CRISPR cassette contains pieces of viral genomes, a genomic “memory” of previous infections. But what was surprising to researchers is that rather than storing remnants of every single virus encountered, bacteria only keep a small portion of what they could hold within their relatively large genomes.
Work published in the Proceedings of the National Academy of Sciences provides a new physical model that explains this phenomenon as a tradeoff between how much memory bacteria can keep versus how efficiently they can respond to new viral infections. Conducted by researchers at the American Physical Society, Max Planck Institute, University of Pennsylvania, and University of Toronto, the model found an optimal size for a bacteria’s immune repertoire and provides fundamental theoretical insights into how CRISPR works.
In recent years, CRISPR has become the go-to biotechnology platform, with the potential to transform medicine and bioengineering. In bacteria, CRISPR is a heritable and adaptive immune system that allows cells to fight viral infections: As bacteria come into contact with viruses, they acquire chunks of viral DNA called spacers that are incorporated into the bacteria’s genome. When the bacteria are attacked by a new virus, spacers are copied from the genome and linked onto molecular machines known as Cas proteins. If the attached sequence matches that of the viral invader, the Cas proteins will destroy the virus.
Bacteria have a different type of immune system than vertebrates, explains senior author Vijay Balasubramanian, but studying bacteria is an opportunity for researchers to learn more about the fundamentals of adaptive immunity. “Bacteria are simpler, so if you want to understand the logic of immune systems, the way to do that would be in bacteria,” he says. “We may be able to understand the statistical principles of effective immunity within the broader question of how to organize an immune system.”
Read more on Penn Today.
Vijay Balasubramanian is the Cathy and Marc Lasry Professor in the Department of Physics and Astronomy in the School of Arts & Sciences at the University of Pennsylvania and a member of the Department of Bioengineering Graduate Group.
This research was supported by the Simons Foundation (Grant 400425) and National Science Foundation Center for the Physics of Biological Function (Grant PHY-1734030).